In the not too . distant future, the Internet of Things will connect several billions of devices to the Internet. This is only possible if cost-effective communications networks and wireless devices are available. By using wireless technologies such as Bluetooth®, ZigBee, Wi-Fi or cellular networks, almost anything, anywhere in the world, can be connected quickly and reliably to the Internet. The latest cellular technologies, eMTC and NB‑IoT, will play an important role in the success of the Internet of Things. These technologies pave already the way to 5G networks of the future optimized not only for enhanced mobile broadband applications but for the diverse IoT applications requiring massive machine type communication (mMTC) or ultra-reliable, low-latency communication (URLLC)
Most applications use local network technologies like Wi-Fi or Bluetooth that operate in license-free frequency bands. In contrast, applications that depend on mobility or global accessibility make use of satellite technologies plus cellular mobile radio technologies, primarily of the second and third generation. Typical examples include fleet management, container tracking, coffee vending machines or ATM services, personal health monitoring, to name just a few.
For the most part, these are applications that generate little data traffic, often needing only an SMS service for transmission. About 86% of today’s cellular IoT devices use second- or third-generation mobile communications technologies. The fourth generation of mobile communications using LTE has played a lesser role to date.
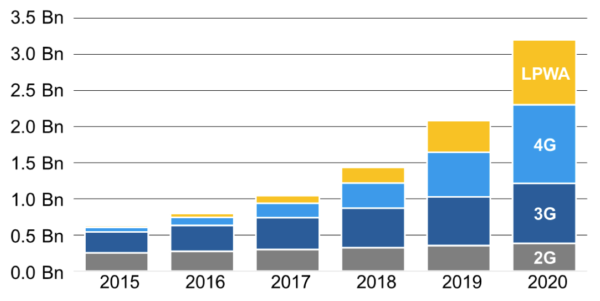
Because LTE is primarily optimized for the mobile broadband market, the IoT has generated little demand for 4G technology. Moreover, the costs for an LTE modem are still relatively high in comparison to a GSM modem. Some aspects of LTE, however, make it increasingly attractive. One of these is global accessibility: According to GSMA, 4G‑LTE networks covered more than a third of the global population by year-end 2015 and by the end of the decade, developed countries are expected to reach ‘full’ coverage.
LTE offers additional technological advantages with respect to spectral efficiency, latency and data throughput. The long-term availability of LTE is another consideration. Second generation networks are in operation for more than 25 years and even though some future evolution provisions have been introduced in the specification, it may be possible that operators will discontinue the service on these networks in long term. Therefore, the industry is looking for LTE solutions being competitive to today’s 2G solutions in terms of cost, power consumption and performance.
Preparing the network for a huge number of connected devices
The need for optimized solutions for the IoT market was also recognized by the 3GPP standardization, and specific improvements for machine type communication (MTC) have been developed. For example, the committee has defined features in Rel. 10/11 intended to protect the mobile network against overload as a result of the many IoT devices. Network operators need to be armed against the possibility of several thousand devices trying to connect to the network at the same time. This could happen after a sudden event, for example the power grid coming back online after a power failure. Overload mechanisms and options for reducing the signaling traffic have been introduced to handle these types of occurrences.
Many IoT applications – sensor networks as an example – only rarely send data and do not need to operate precisely to the second. These devices can report to the network that they are prepared to accept longer delays during the connection setup (delay tolerant access). Rel. 10 includes a process that permits the network to initially reject the connection requests from these devices and delay them until a later time (extended wait time). With Rel. 11, access to the cellular network can be controlled by means of access classes. In this case, a device may set up a connection only if it is assigned a class that is currently permitted by the network. The network transmits a bitmap (EAB barring bitmap) that identifies which classes are permitted access.
These processes introduced in Rel. 10 and 11 ensure reliable and stable operation of the IoT applications and devices of today and tomorrow within cellular networks without endangering the mobile broadband service.
Low power, low complexity modems
The only thing still missing were optimized solutions for IoT devices with low data traffic, low power consumption and low costs. The committee started on those in Rel. 12. It quickly became clear, however, that there will be no single, simple solution for all applications. The requirements for applications such as container tracking, waste bin management, smart meters, agricultural sensors and sports and personal health trackers are too varied. Rel. 12 therefore concentrates on the areas of power consumption and cost-effective modems. The results are a power-saving mode (PSM) that is especially important for battery-operated devices and a new LTE device category 0, which should have only 50 % of the complexity of a category 1 modem.
The PSM process starts after a data link is terminated or after the periodic tracking area update (TAU) procedure completes. The device first goes into the idle mode in which it periodically switches to receive mode in order to receive messages (discontinuous reception). As a result, it remains reachable via paging. After timer T3324 expires, the power saving mode is entered. In this mode, the device is always ready to send messages because it remains registered in the network.
However, the receiver is literally switched off so the device is not accessible via paging. PSM is thus suited for sensor networks that only rarely need to send data to the device. This mode is not suitable for applications that require a quick response from the sensor or expect a time-critical reaction. Applications that use PSM must tolerate this behavior and the design process must include the specification of optimal timer values for idle mode and power-saving mode.
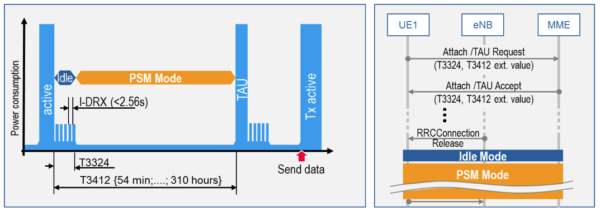
The introduction of category 0 was a first attempt at permitting significantly less expensive LTE modems for the M2M market. Ideally, they will also use less power. To achieve this, the complexity of the modem was reduced by bringing the supported data rate down to 1 Mbps. This minimizes the requirements for processing power and memory. Manufacturers can also eliminate full duplex mode and multiple antennas. As a result, the device does not require duplex filters that otherwise would be necessary to prevent interference between the transmitter and receiver.
Cellular low power WAN networks
LTE category 0 was the immediate step towards LTE category M1 introduced in Rel 13. With category M1 additional cost-reduction measures, especially lower bandwidths in the uplink and downlink, lower data rates and reduced transmit power were implemented.
A new standard called narrowband IoT (NB-IoT) was developed in parallel with LTE category M1. The requirements profile for this standard includes extremely low power consumption, very low costs, improved reception in buildings and support for an enormous number of devices with very little data traffic. NB-IoT has a bandwidth of just 180 kHz and can be deployed by using unused LTE resource blocks, free spectrum between neighboring LTE carriers (guard band) or stand-alone, for example in unused GSM carriers. With the NB-IoT 3GPP has created a new cellular air interface that is fully adapted to the requirements of typical machine type communications.
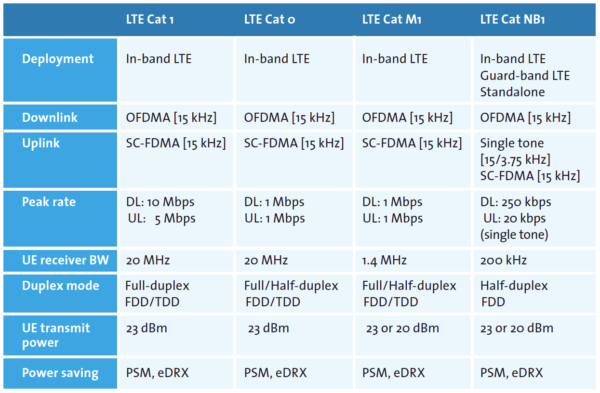
An additional feature for reduction of the power consumption was implemented as well. With extended DRX (eDRX) the time interval is extended the modem goes into receive mode to receive paging messages and system status information. The DRX timer determines how often this occurs.
Currently, the shortest interval for the Idle DRX timer is 2.56 seconds. That is fairly frequent for a device that expects data only every 15 minutes and has relaxed delay requirements, for example. With eDRX the timer can be configured now in the range from some few seconds to some hours.
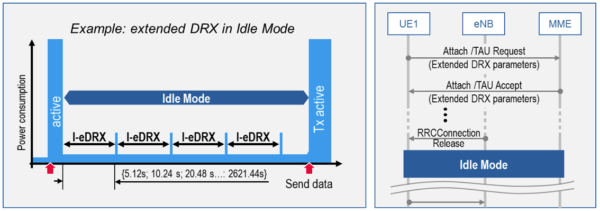
The main differences between PSM and eDRX are the allowed time staying in a kind of power off mode and the procedure to switch into receive mode. A device using PSM mode needs to go first in the active mode to become reachable and stays afterwards for a certain time in idle mode. A device using eDRX can stay in the idle mode and go just quickly in the receiver mode, without any additional signaling.
There is also a need to improve the coverage for applications involving smart meters installed under extreme receive conditions, such in a basement, or for widely dispersed devices, such as in agricultural applications. Techniques like redundant transmission, power sensitivity boosting, frequency hopping or the use of lower order modulations are used to significantly improve the coverage.
On the way to 5G networks
3GPP is actually working on a couple of topics to further improve LTE networks for the Internet of Things, examples are enhanced NB-IoT (eNB-IoT) with new power classes, improved mobility support and multicast messaging, or further enhancements for eMTC (feMTC) including VoLTE support and multicast messaging. These kind of enhancements are the next steps in the direction of 5G network for massive MTC (mMTC).
But there is a second category of IoT applications requiring ultra-reliable and low latency connectivity. 3GPP is working on these aspects as well with adopting D2D communications for vehicle to vehicle communication, or general latency improvements for example.
THINGs are different
Connected things are different in several aspects. As described above they are connected via technologies optimized for the IoT use cases. But they are also different in the aspects like ‘customer experience’. A smart meter isn’t able to run outside and search for better network coverage. It is difficult to re-charge the battery of a parking slot sensor. These kind of sensor should work several years without need for maintenance. And probably most important 24/7 availability of each single THING is a must especially in case of IoT applications in the areas of healthcare, industry automation, or smart cities.
Joerg Koepp, Rohde & Schwarz