Abstract — . The technical development towards the full vision of the Internet of Things (ioT) is expected to reach it´s full potential by the coming 5G wireless technologies. This will open up for so called massive IoT characterized by co-location densities in the order of 200000 devices per km2. This development will affect the area of Electromagnetic Compatibility (EMC) in a number of ways and may even be the most challenging issue for the EMC area since it was born for over 100 years ago. In this paper, some of these new challenges are highlighted and discussed. Furthermore, numerical results from some dense co-location scenarios are presented to show examples of interference challenges to be handled.
.
I. INTRODUCTION
The development towards the vision of the so-called Networked Society, driven by the Internet of Things (IoT) is going faster for every year. The term IoT refers to the internetworking of physical devices, vehicles buildings and other items – embedded with electronics, software, sensors, actuators, and network connectivity that enable these objects to collect and exchange data. Kevin Ashton, one of the founders of the original Auto-ID Center, is usually considered as the first to use “Internet of Things” in a wider sense in 1999.
The 5G vision in the telecommunication industry is today seen as the necessary technical enabler to really make IoT happen at full scale. 5G is being designed as the key enabler of the future digital world, where ubiquitous ultra-high broadband infrastructure will support the transformation of processes in all economic sectors and meet the growing consumer market demand. The wireless part of global Internet traffic is expected to grow from approximately 50% today, to about 75% in 2020, and the first 5G products are expected to be available in a larger amount 2020. Instead of the World Wide Web (WWW) we are therefore now seeing the World Wide Wireless Web (WWWW). The 5G development is therefore expected to pave the way for ultra-high co-location densities denoted as massive IoT applications. Massive IoT applications refer to co-location densities in the order of 200 000 devices per km².
The overall goal of Electromagnetic Compatibility (EMC) is the correct operation of different equipment in a common electromagnetic environment. The common way to achieve this goal is today typically solved by a pure technical engineering approach where standards, regulations and proven design criteria play a fundamental role. This approach may in most cases achieve EMC, at least for static cases and scenarios that do not change significantly over time. However, the more unpredictable scenarios we have, the more difficulties this static approach will experience. The current development towards massive IoT will lead to a massive increase of wireless technology and dynamic scenarios and this will give several new challenges for the EMC area. Several technical changes that affects EMC issues will occur in this development such as
- considerably higher densities of co-located wireless consumer devices,
- dynamic unpredictable co-location scenarios,
- new frequency bands, with unknown immunity and radiated properties for the products,
- considerably higher frequencies as well as larger bandwidths than present EMC testing is performed for,
- an increased use of off-the-shelf RF (radio frequency) modules integrated in products,
- an increased use of cheap wireless technologies in safety & security applications as well as autonomous vehicles and medical applications.
The increased co-location densities of devices will lead to a need of further development of the overall methodology of achieving EMC. The increased use of new (higher) frequency bands and larger bandwidths will require a further development of EMC test equipment and methodology as well as investigations of immunity and radiated properties of the new IoT products. We can already today see an increased use of off-the-shelf RF modules in product development and this will increase in IoT products. To avoid introduction of new EMC-problems, this requires a careful EMC integration methodology since such RF-modules are developed to function under the assumption of certain specific technical parameters such as antennas, frequency bands, duty cycles, grounding principles, power supplies and necessary physical distance to metal structures.
Without EMC this massive increase of wireless products in dynamic and dense co-location scenarios will be limited in performance. It is therefore likely to assume that EMC will be a crucial area for getting the full business potential out of massive IoT. The overall conclusion of these technical trends is that the EMC area probably will undergo the largest development since it was born for over hundred years ago. This development could be expected to be very exciting and vitalizing for the whole EMC area and in this paper we will give some more concrete examples of these expected challenges.
The paper is organized as follows. In section II possible wireless candidates for different IoT applications are described and some numbers of expected co-location densities and duty cycles for IoT-products are shown and the consequences of these are discussed. In section III, some numerical results of the increased co-location densities and duty cycles are shown. The conclusion is that some of these scenarios may cause severe interference problems and therefore have to be handled with great care for EMC issues, not to limit the full business potential for massive IoT. The paper is concluded in section IV.
II. TECHNICAL PARAMETERS FOR IOT
A. Connected devices
Today, there exists a plethora of wireless candidates for IoT applications. In Fig. 1. An overview of some key candidates is shown.
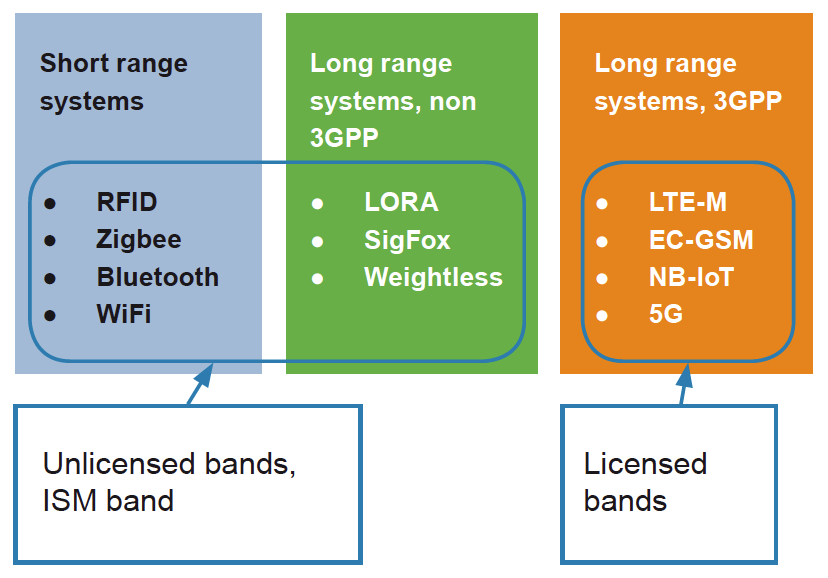
It should be noted that The 5G development also targets applications in unlicensed spectrum, even if the main target is licensed spectrum. This development within IoT will highlight several new challenges for EMC. The vision of the IoT involves, in principle, all sectors of society. Examples are smart cities, e-health, smart homes, smart grids, smart agriculture, intelligent transport systems (ITS), logistics, industrial control, environmental monitoring, education, entertainment and media [1].
Forecasts of the growth of the number of connected devices are done continuously by different actors. A few years ago, forecasts were in the order 50 billion devices 2020, but those forecasts have now been considered as too optimistic [2]. Fig. 1 shows a typical example of current forecasts. The largest growth in Fig. 2 is due to IoT-applications whereas the growth of PC/laptop/tablets and mobile phones typically is estimated only to a few percent from 2017 to 2023. This development will create a complexity of co-location scenarios that has never been seen before.
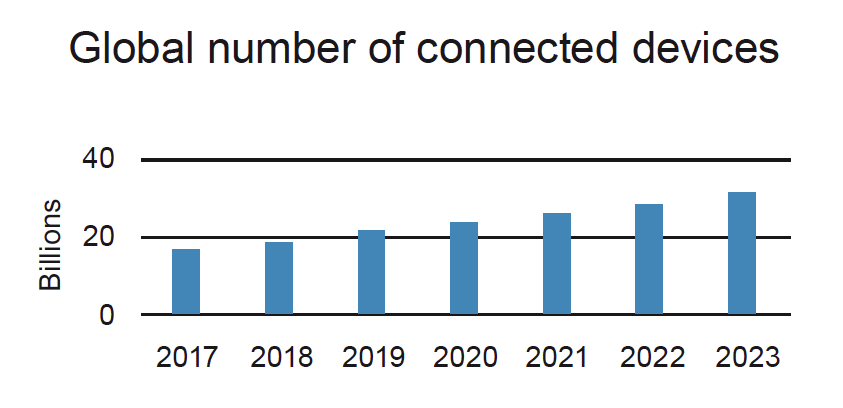
B. Co-location densities and massive IoT
New electronic devices, both non-wireless and wireless will be co-located almost everywhere in the society and these scenarios will be highly random and dynamic, strongly affected by the habits of the consumers. The most dense scenarios are denoted massive IoT and will be characterized by being highly dense and of large complexity from an interference point of view. An example of massive IoT is machine-to-machine (M2M) applications with up to 200 000 devices per km². Another application area for massive IoT is smart cities. Predictions of the number of co-located devices per area unit says up to 10 000/km². for massive IoT connected devices in dense smart cities [3], see Table 1.
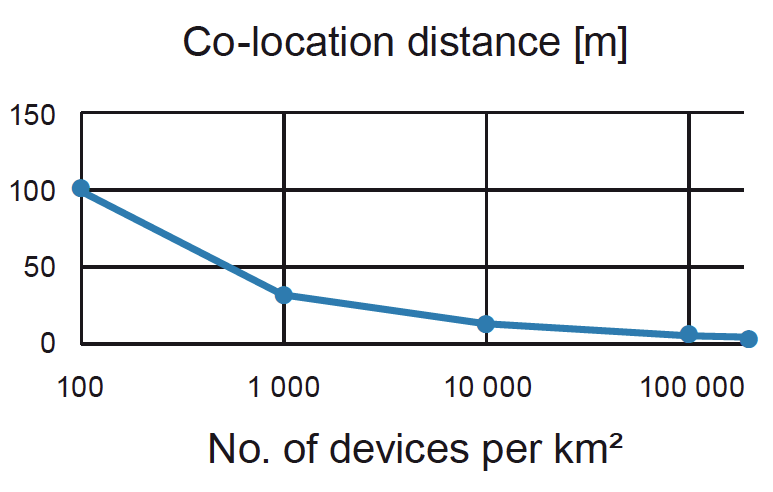
Co-location distances between wireless receivers and electronics fulfilling different standard radiated emission limits have been determined in several publications, e.g. [6]. The minimum co-location distance to avoid a large risk for interference problems is highly dependent on the assumptions on e.g. interference waveform, signal-to-noise ratio for the wireless receiver and the emission standard considered. However, results from such analyses varies between a few meters up to hundreds of meters. Thus, a rough analysis shows that the range of such minimum co-location distances fall in the interval of expected co-location densities of connected devices in massive IoT scenarios. This indicates that these higher co-location densities will be challenging from an EMC point of view.
C. Duty cycles
The duty cycles, α, in IoT products will vary largely depending on application. In some applications, energy saving are of primary concern and therefore as low duty cycles as possible are used. In other applications, real-time requirements require higher duty cycles to be used. In Table 2, examples of the variety of duty cycles in IoT products are shown. As seen in Table 2, duty cycles can vary over a large span which in turn affects the co-location properties in dense scenarios.
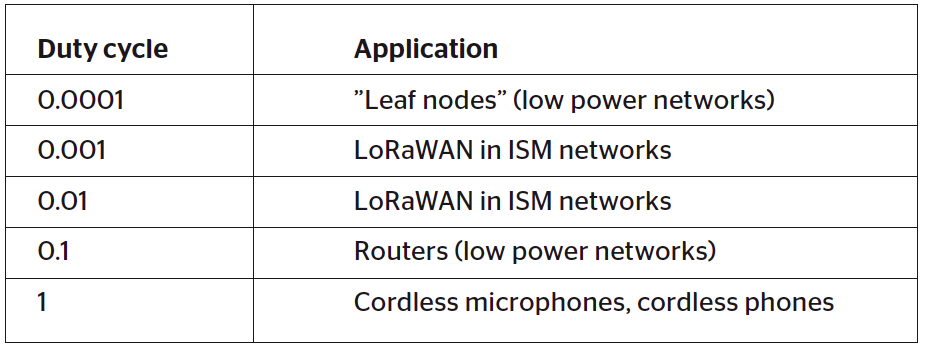
III. EXAMPLES OF INTERFERENCE LEVELS IN MASSIVE IOT SCENARIOS
As indicated earlier, the introduction of a high density of devices will affect the EM environment. In the following analysis, the ISM frequency band 5,8 GHz MHz is investigated. Although the densities are very high, the total interference contribution from the devices will be affected by the duty cycle of the system. As could be seen in Table 3, many of the applications are characterized by very low values of the duty cycle. The duty cycle impacts the result in the way that it is a certain probability that the device is transmitting.

The device density is assumed to be either 10 000 devices per square kilometer or 200 000 devices per square kilometer, where the latter case may be appropriate to model crowded areas, such as in-house or in business centers. In this frequency band, different options of transmitting power are occurring and in Table 3, the assumed value for the analysis is shown The devices are assumed to be randomly located in an area of 100 times 100 meters. The adopted densities implies that there will be 100 and 2000, in average in the area.
The antenna is assumed to be isotropic with 0 dB in gain. By assuming free-space path loss model for the wave propagation of the emission from the IoT device, the contribution to the overall electromagnetic environment can be estimated. The free-space path loss is reasonable since the distances are short. The duty cycle impacts the result in the way that it is a certain probability that the device is transmitting.
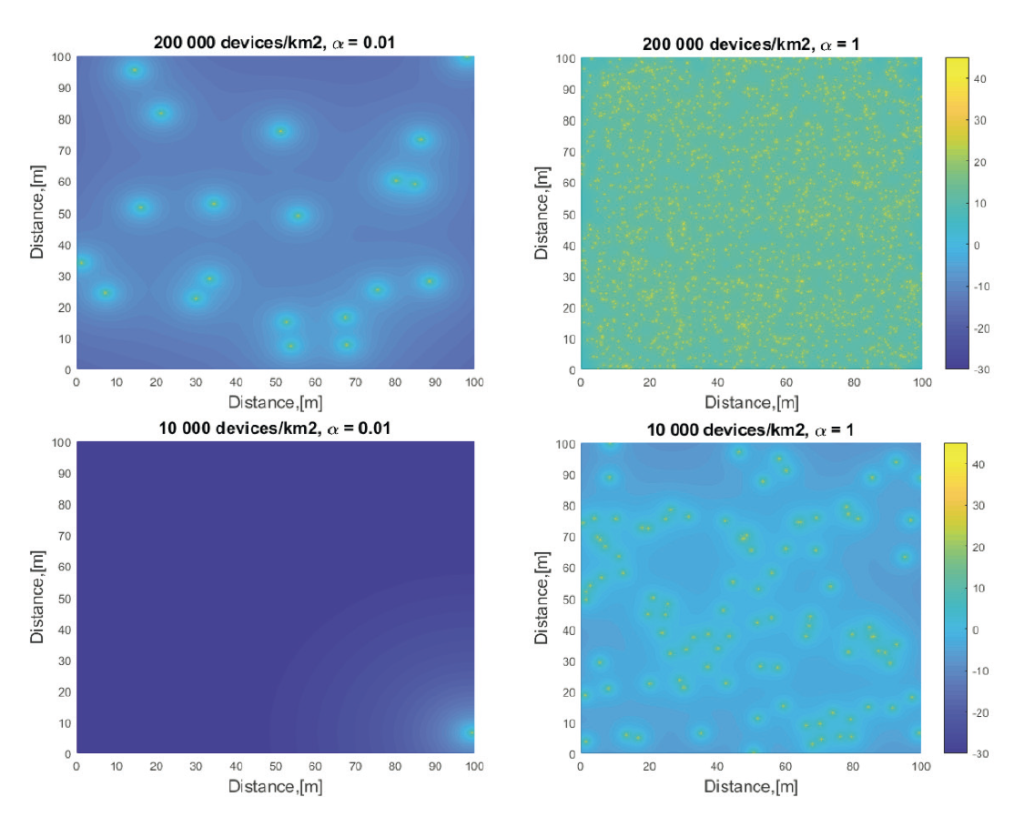
In Fig. 4, the contribution to the received power from the devices is calculated in a square of 100 m x 100 m, when the transmitter power is 200 mW at 5.8 GHz. In the figure, a snapshot of the experienced power in dBμW is plotted for different device densities and duty cycles. It is evident that the duty cycle and the density are highly affecting the received power. In the more dense scenario, with 200 000 devices per square kilometer, the power levels in the areas is substantially higher. For 200 000 devices/km². and a duty cycle of 1 it can be seen that the interference power is often exceeding the level of 53 dBuW, which is the transmit power of the devices. In this case, the total interference power is a result of many IoT devices. For a density of 10 000 devices/km², the level is more often around the transmit power and lower.
In Fig. 5, it is possible to more clearly analyze the interference level. The figure shows the estimated cumulative density function (cdf) of the received power.
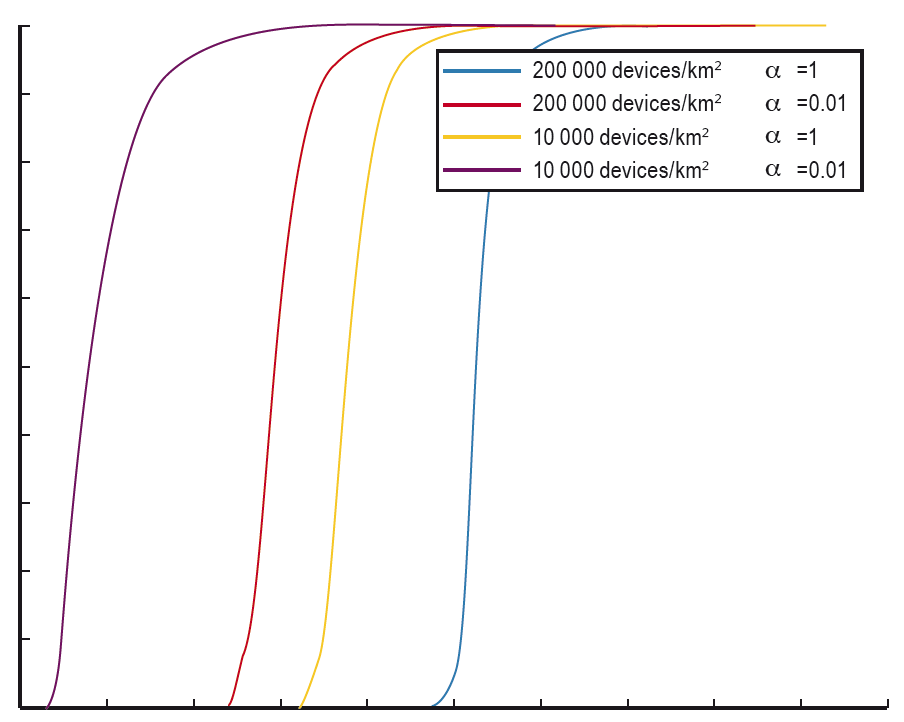
In Table 4, the interference power over the receiver noise in dB is summarized for a receiver operating with 25 kHz. The exceedance of the thermal receiver noise is determined in dB for a probability of 50 % and a noise figure of 15 dB. It can be seen that the noise floor is exceeded in all the cases with a significant amount.
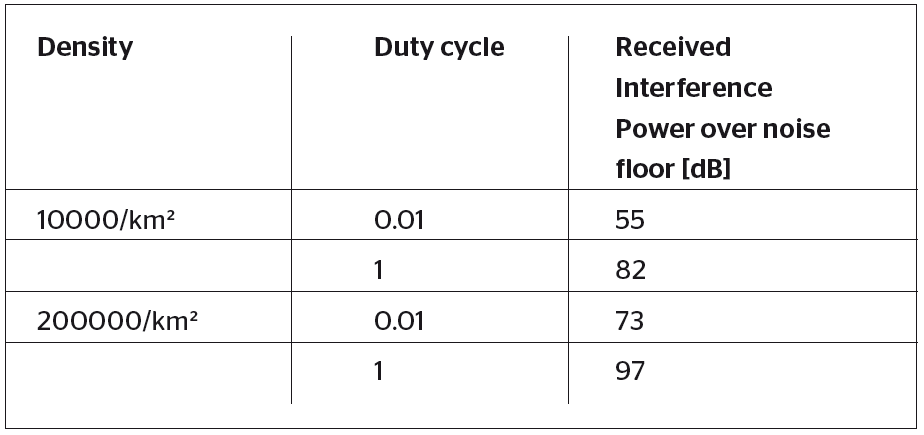
IV. CONCLUSIONS
The development towards massive IoT will create several new challenges for the EMC area. Some of the foreseen dense co-located scenarios may cause severe interference problems and therefore have to be handled with great care for EMC issues, not to limit the full business potential for massive IoT. These challenges will probably develop the EMC area in an exciting and vitalizing way.
Kia Wiklundh, Qamcom Research & Technology Peter Stenumgaard Swedish Defence Research Agency (FOI) References
[1] V. Angelakis, E. Tragos, H. C. Pöhls, A. Kapovits, A. Bassi, Designing, Developing, and Facilitating Smart Cities: Urban Design to IoT Solutions, Springer, 2017. [2] Amy Nordrum, “Popular Internet of Things Forecast of 50 Billion Devices by 2020 Is Outdated”, IEEE Spectrum, Aug 2016. [3] “Ericsson Mobility Report”, November 2017. [4] Ericsson Technology Review, Vol. 93, no. 3, 2016. [5] P Stenumgaard, K Fors, K Wiklundh, ”Interference Impact on LTE from Radiated Emission Limits”, Proceedings of IEEE EMC 2015, Dresden, Germany. [6] Kia Wiklundh, Peter Stenumgaard, “EMC Challenges of the Internet of Things“, Electronic Environment, no. 1, 2017, pp. 13-15.